
HYPERGONADOTROPIC HYPOGONADISM,
PROGRESSIVE EARLY-ONSET SPINOCEREBELLAR ATAXIA,
AND LATE-ONSET SENSORINEURAL HEARING LOSS:
CASE REPORT AND LITERATURE REVIEW Sarikaya E,1 Ensert CG,2 Gulerman HC1 *Corresponding Author: Esma Sarikaya, Centre for Reproductive Medicine, Zekai Tahir Burak Women’s
Health Research and Education Hospital, Talatpasa Bulvari Hamamonu 06230, Ankara, Turkey; Tel.: +90-312-
310-3100; Fax: +90-312-312-4931; E-mail: sudesarikaya@hotmail.com page: 77
|
DIFFERENTIAL DIAGNOSIS
Phenotypic overlap and inter familial variability
make the hereditary ataxias a notoriously difficult
group of disorders to classify. New gene loci, which
are linked to SCAs, are discovered every other day.
Endocrine dysfunction is a common clinical feature
in these patients, with hypogonadism occurring in approximately
5% [26]. The same problem is also present
for SNHL, which is a component of more than 400
syndromes, and, so far, more than 30 genes associated
with syndromic hearing loss have been identified. To
date, more than 70 loci have been reported for non
syndromic deafness, including approximately 40 autosomal
dominant, 30 autosomal recessive, and eight Xlinked,
and approximately 30 genes have been cloned.
Mutations in GJB2, the gene that encodes the connexin
26 protein, are the most common cause of recessive
deafness in the United States, and dominant low-frequency
SNHL is most commonly caused by mutations
in the Wolfram’s syndrome 1 gene. Usher syndrome
is the most frequent cause of combined deafness and
blindness in the industrialized world [27].
Association of ataxia and hypergonadotropic hypogonadism
and hearing loss is extremely rare and
part of well described autosomal recessive syndromes
other than PS [6-9] (Table 1). Other syndromes that
exhibit two or more signs of AAHH are: trinucleotide
repeats disorders; fragile X syndrome (CGG repeat),
Friedreich’s ataxia (GAA repeat) and five SCAs (1, 2,
3, 6 and 7) (CAG repeat), myotonic dystrophy (DM/
Curschmann-Steinert disease), proximal myotonic
myopathy (PROMM or Ricker’s syndrome) (CTG
repeats) [28], ciliopathies; Alström syndrome, Bardet-
Biedl syndrome [29], the neurodegenerative mitochondriopathies;
Huntington’s disease, Charcot-Marie-
Tooth (CMT), Leber’s hereditary optic neuropathy,
Kearns-Sayre syndrome (KSS), maternally inherited
Leigh syndrome (MILS), and the neuropathy, ataxia,
and retinitis pigmentosa (NARP) syndrome and other
mitochondriopathies; Wolfram’s syndrome [30],
muscle lipid diseases; lipid storage myopathy (LSM),
multiple acyl-CoA dehydrogenase deficiency, glutaric
aciduria type II, and neutral lipid storage disease with
myopathy, β-oxidation cycle defects and deficiencies
of carnitine palmitoyltransferase II (CPTH) and very
long chain acyl-CoA dehydrogenase (VLCAD) [31].
Refsum’s disease (phytanic acid storage disease),
and Zellweger syndrome (cerebro-hepato-renal syndrome),
Uscher, Alport, Cockayne, Flynn-Aird, Hurler
(MPS-1), Kearns-Sayre (CPEO), Norrie, 4H (hypomyelination
with hypogonadotropic hypogonadism and
hypodontia), Sohval-Soffer, Crandall, Woodhouse-
Sakati, Marinesco-Sjögren’s, CAPOS syndromes, galactosemia
and hereditary motor sensory neuropathies
(HSMN)[16,23,33-38].
In a few reports, several moleculer analyses have
been made for the differential diagnosis of these patients:
Pierce et al. [5] applied whole-exome sequencing to identify the gene responsible for PS and found
mutations in 17β-hydroxysteroid dehydrogenase type
4 [also known as D-bifunctional protein (HSD17B4/
DBP)], which is also detected in Zellweger syndrome
[23]. They have also found mutations in mitochondrial
histidyl tRNA synthetase HARS2 [38]. Nishi et al.
[10] found no evidence of mitochondrial disease on a
muscle biopsy, including respiratory enzyme assays;
no ragged red fibers were found on muscle biopsy and
there was only mild type IIB atrophy, a non specifc
finding in many types of upper motor neuron lesions.
In the evaluation by Fiumara et al. [16] none of the
known mitochondrial or CMT mutations were present
in the DNA of patient 1. Muscle biopsy showed atrophy
with fat replacement. They also performed sural
nerve biopsy in one patient (SM) and found severe
loss of myelinated fibers with segmental demyelination
and remyelination and scattered axonal degeneration
suggestive of a form of hereditary motor sensory
neuropathy (HSMN). Mitochondrial testing on patient
1 (LK) for MELAS 3243, SNHL-1555 and -7445 also
did not show any of the mutations known to cause
the mitochondrial encephalomyopathy, lactic acidosis,
and stroke-like episodes or some form of SNHL
[16]. Marlin et al. [19] sequenced the whole mitochondrial
genome, GJB2 (the most frequent form of
isolated hearing impairment), AOA1, POLG, FRDA,
(genes implicated in ataxia or ophthalmoplegia) and
FOXL2 (a gene responsible for a premature ovarian
failure), and did not find any mutation in patient 2. In
one female with this association, partial deficiency of
the mitochondrial enzyme cytochrome c oxidase was
demonstrated by De Michele et al. [12]. Gironi et al.
[39] reported two brothers who had late-onset progressive
ataxia and hypergonadotropic hypogonadism associated
with muscle CoQ10 deficiency.
Before starting further laboratory investigations,
we think clinical and neurological examinations must
be fully performed in such cases. Long-term follow up
is very important in these patients since some clinical
manifestations appear later in life. It is also important
to collect details on the general health, hearing, menstrual
history and to report the presence of morphologic
abnormalities of the ear, eye, face, or other organ
systems, mental retardation, ataxia, epilepsy, Parkinson’s
disease, autism of the relatives from both parental
lines. It is also relevant to check for consanguinity and
family ethnic background. This simple approach will
provide the opportunity to recognize associated syndromes
and evidence of the basis behind the initiation
of further laboratory investigations. The assessment
of a patient with AAHH requires a multidisciplinary
approach and must include advice and support to the
parents, and genetic counseling aimed at optimizing
the use of the most adequate clinical resources. The
laboratory tests must be confirmed according to clinical
suspicion. Before making costly molecular analyses,
simple blood tests for glucose, vitamin E, folate
and B12 levels, α-fetoprotein, very long chain fatty
acids and phytanic acid, lysosomal enzymes, amino
and organic acids serum ammonia, arterial pH levels,
and X-rays of the skeleton should be done. Performing
ECG, muscle biopsy, measurement of mitochondrial
enzyme cytochrome c oxidase and muscular CoQ10
levels and DNA analysis for trinucleotide expansions
at the SCA 1, 2, 3, 6, 7 and Friedreich’s ataxia loci, and
looking for mutations in HSD17B4 for a number of
similar cases will give valuable information about the
pathogenesis and differential diagnosis of this association.
The late-onset of SNHL in many reported cases
underlines the need for careful examination of acoustic
acuity in cases of cerebellar ataxia and hypogonadism.
The audiograms are also important to guide patient
rehabilitation.
The pathogenetic mechanism of AAHH is not yet
fully understood. Considerable heterogeneity exists
in the literature on the neurological manifestations,
onset age, progression of symptoms, clinical severity
and presence of associated abnormalities such as
mental retardation (PS, Richards-Rundle syndrome)
[5,11-15,19], epilepsy (mitochondriopathies) [12,14],
nystagmus [3,10,12,19, present study], cataract, optic
atrophy, retinal dystrophy or blindness (Alström and
Wolfram’s syndrome, mitochondriopathies) [14,15],
and endocrine anomalies (Wolfram’s, Alström, Richards-
Rundle syndromes, mitochondriopathies) [10],
short stature (Alström syndrome) [5,10], cardiac anomalies
(PS and mitochondriopathies) in this association.
This heterogeneity may be reflecting different degrees
of severity within the entity of ovarian dysgenesis and
neurological impairment and favors the hypothesis that
AAHH is a heterogeneous syndrome. It is therefore
very unlikely that cerebellar ataxia and hypogonadism
are linked to a common genetic defect. As the physiopathology
of PS is still unknown, the question remains
whether one gene is implicated in all the different
clinical forms of PS (with and without neurological
symptoms) or if there are at least two different genes responsible for two different clinical entities. It is more
reasonable to ascribe this association as a clinical entity
with a different, yet not fully understood, pathogenetic
mechanism in which the involvement of different
genotypes may lead to the same or similar phenotypic
manifestations. Association of ataxia hypergonadotropic
hypogonadism and hearing loss could be caused by
both a malfunction of the mitochondria and of myelination.
Depending on the genes involved, it may have
autosomal recessive or mitochondrial inheritance. The
inter familial variation of both the severity of hearing
loss and the onset of amenorrhea, and neurological
symptoms in individuals with AAHH may turn out to
be due to environmental or genetic factors influencing
the effect of mutations.
Proposed Causative Pathogenic Mechanisms
Were as Follows:
1) Polymorphisms within the mitochondrial genome
might lead to impaired energy generation and
to an increased amount of reactive oxygen species,
having susceptibility in several diseases. Mitochondria
produce most of the cell’s supply of adenosine triphosphate
(ATP), by means of the oxidative phosphorylation
machinery, which comprises electron transport
chain (ETC) and ATP synthase. The ETC dysfunction
leads to reduced ATP production, impaired calcium
buffering and increased generation of reactive oxygen
species (ROS). Mitochondria have their own DNA;
the mitochondrial DNA (mtDNA) carries 37 genes: 22
encoding for mitochondrial transfer RNAs (tRNAs)
(for the 20 standard amino acids, plus an extra gene for
leucine and serine), two for ribosomal RNAs (rRNAs)
and 13 encoding for polypeptides subunits of complexes
of the respiratory chain system, seven of these
belong to complex I or NADH dehydrogenase (ND1,
ND2, ND3, ND4, ND4L, ND5, ND6), one to complex
III or cytochrome c reductase, three to complex IV or
cytochrome c oxidase (COX I, COX II and COX III)
and two to complex V or ATP synthase (ATPase6 and
ATPase8). Increased production of ROS damages cell
membranes and further accelerates the high mutation
rate of mtDNA. Mitochondrial genetics differs from
Mendelian genetics in three major aspects: maternal
inheritance, heteroplasmy and mitotic segregation.
Mitochondria are inherited in humans via the female
line, transmitted as a non recombining unit by maternal
inheritance [40].
There is significant evidence that the pathogenesis
of several neurodegenerative diseases, including
Parkinson’s disease, Alzheimer’s disease, Friedreich’s
ataxia, multiple sclerosis and amyotrophic lateral sclerosis,
may involve the generation of reactive oxygen
species and/or reactive nitrogen species associated
with mitochondrial dysfunction [40]. Leipnitz et al.
[41] reported that oxidative stress is elicited in vitro
by phytanic acid (Phyt) that accumulates in Refsum
disease and other peroxisomal diseases. Phytanic
acid increased thiobarbituric acid-reactive substances
(TBA-RS) levels, carbonyl content and sulfhydryl
oxidation, and decreased concentrations of glutathione
(GSH). This effect was prevented by the antioxidants
α-tocopherol and melatonin, suggesting the involvement
of free radicals [41].
In one female with this association, partial deficiency
of the mitochondrial enzyme cytochrome c
oxidase was demonstrated by De Michele et al. [12].
Primary MCPs are either due to sporadic or inherited
mutations in nuclear or mitochondrial DNA located
genes. The MCPs should be considered in any patient
with unexplained progressive multi system disorder in
addition to AAHH [9].
2) It can be related to the defect of a gene producing
a protein or other product that affects both
granulosa cells, neurons and hearing function. Proteins
mentioned in the literature were wolframin, usherin,
myosin, harmonin, cadherin 23, protocadherin
15, SANS, whirlin, VLGR1b Clarin-1, D-bifunctional
protein (DBP) GPR98 proteins, connexin 26, neuronal
proteins, mitochondrial protein, protein zero, norrin,
huntington, frataxin, MKS1 and meckelin.
In fragile X-associated tremor/ataxia syndrome
(FXTAS), which is one of the most common singlegene
forms of gait ataxia and tremor in older males;
pre mutation in FMR1 causes the gene to make abnormally
increased amounts of messenger RNA. This
can be toxic to certain brain cells. A similar toxic
mechanism might impair ovarian function and lead to
hypergonadotropic hypogonadism [42]. Mutations in
the SIL1 gene on chromosome 5q31 cause Marinesco-
Sjögren’s syndrome and the loss of SIL1 function
results in accumulation of unfolded proteins that are
harmful to the cell [43].
Mutations in the GALE genes on chromosome
1p36-p35, GALK1 genes on chromosome 17q24,
and GALT genes on chromosome 9p13 cause galactosemia.
In galactosemia, hypergonadotropic hypogonadism
may be due to toxic effect of galactose or its
metabolites to the ovarian parenchyma [44]. Friedreich’s ataxia and Huntington’s disease are
classified in the neurodegenerative mitochondriopathies
and trinucleotide repeat disorders. Friedreich’s
ataxia is caused by a GAA repeat expansion and mutations
in the FXN gene on chromosome 9 that encodes
frataxin. Frataxin is a mitochondrially targeted protein
that is an iron chaperone and plays a role in mitochondrial
iron handling which requires ETC constituents
[45,46].
Huntington’s disease is caused by a CAG repeat
expansion in one copy of the Huntingtin gene on chromosome
4, which encodes a protein called huntingtin
that has been shown to physically associate with mitochondrial
membranes and interfere with mitochondrial
calcium handling. Huntingtin has been proposed to
interfere with mitochondrial biogenesis by disrupting
peroxisome proliferator activated receptor γ coactivator
1 α (PGC-1a), a transcription co-activator that facilitates
mitochondrial biogenesis [47,48].
A mutation in the NDP gene causes Norrie disease.
This gene encodes a secreted protein with a cysteinknot
motif that activates the Wnt/β-catenin pathway.
The protein forms disulfide-linked oligomers in the extracellular
matrix. For the normal development of the
eye and other body systems, norrin is believed to be
crucial. Norrin also appears to be crucial in the specialization
of the cells of the retina and the establishment
of a blood supply to the inner ear and the tissues of the
retina [49].
Mutations in HSD17B4 type 4 leads to a fatal
form of Zellweger syndrome and may be PS [5,23]. Dbifunctional
protein (DBP) and multifunctional protein
2 (MFP-2) encoded by HSD17B4, is a multi functional
peroxisomal enzyme involved in fatty acid β-oxidation
and steroid metabolism [5,23].
The WFS1 or wolframin gene provides instructions
for making the wolframin protein which is located
in the endoplasmic reticulum. More than 30 WFS1
mutations have been identified in individuals with a
form of non syndromic deafness called DFNA6. Researchers
have identified more than 100 WFS1 mutations
that cause Wolfram syndrome. Some mutations
delete or insert DNA from the WFS1 gene. As a result,
little or no wolframin is present in cells. Other mutations
replace one of the amino acids used to make wolframin.
These mutations appear to reduce wolframin
activity dramatically [50].
The USH2A gene encodes usherin protein and
possesses laminin epidermal growth factor as well as
fibronectin type III domains. Laminins are the major
non collagenous components of basement membranes
that mediate cell adhesion, growth, migration
and differentiation. The discovery of three putative
missense mutations (C319Y, N346H and C419F) in
the laminin type VI domain of this protein mark this
region for a potentially significant functional role in
the cochlea and retina. Netrins are small diffusable
proteins that control guidance of central nervous system
commissural axons at the midline and peripheral
motor axons. A homolog of netrin in C. elegans,
UNC-6, is one of the cues in the extracellular matrix
that guides dorsoventral migrations of pioneer axons
and migrating cells along the body wall on the epidermis
[51].
Mutations in 35delG, GJB2, the gene that encodes
the connexin 26 protein a gap junction protein that is
assumed to be a component of the potassium recycling
pathway in the inner ear, are the most common cause
of recessive deafness in the United States. To date, 48
recessive and seven dominant disease-causing GJB2
mutations have been identified in the 35delG allele,
and is particularly common in Caucasian populations
[52].
Charcot-Marie-Tooth disease (when associated
with essential tremor and ataxia, called Roussy-Levy
syndrome), which is the most common inherited neurological
disorder, is caused by mutations that cause
defects in neuronal proteins. The most common cause
of CMT (70-80% of cases) is the duplication of a large
region in chromosome 17p12 that includes the gene
PMP22. Some mutations affect the gene MFN2, which
codes for a mitochondrial protein [53].
3) An inherited unstable trinucleotide repeat
disorder, which is caused by an expansion of repetitive
three bases in the causative gene, likely shares a
common pathogenesis caused by the gain of a toxic
function of the expanded polyglutamine tract and neurodegeneration.
Fragile X syndrome (CGG repeat),
Friedreich’s ataxia (GAA repeat) and five SCAs (1, 2,
3, 6 and 7) (CAG repeat), myotonic dystrophy (DM/
Curschmann-Steinert disease), proximal myotonic
myopathy (PROMM or Ricker’s syndrome) (CTG repeats)
Huntington’s disease (CAG repeat), major psychosis
[28,54].
Recent findings in genetic research have suggested
that a large number of genetic disorders, both
genetic syndromes and genetic diseases, that were not
previously identified in the medical literature as related, may, in fact be related in the gene-typical root
cause of the widely-varying, phenotypically-observed
disorders.
Mutations in HSD17B4 (also known as D-bifunctional
protein), which leads to a fatal form of Zellweger
syndrome, have recently also been proposed
as the first identified genetic cause of PS by Pierce et
al. [5] and de Launoit et al. [23]. Alström syndrome
is a ciliopathy [29]. Other known ciliopathies include
primary ciliary dyskinesia, Bardet-Biedl syndrome,
polycystic kidney and liver disease, nephronophthisis,
Meckel-Gruber syndrome and some forms of retinal
degeneration [55].
Gempel et al. [25] reported that the electron transferring
flavoprotein dehydrogenase (ETFDH) gene,
previously shown to result in another metabolic disorder,
glutaric aciduria type II (GAII), leads to a secondary
CoQ10 deficiency. Their results indicated that
the late-onset form of GAII and the myopathic form of
CoQ10 deficiency are allelic diseases.
Aguglia et al. [56], described two brothers with
Marinesco-Sjögren’s syndrome, both of whom also
had very low serum vitamin E concentrations with
an absence of postprandial chylomicrons. Findings
on electron microscopy of the intestinal mucosa were
consistent with a chylomicron retention disease. They
also suggested that both chylomicron retention disease
and Marinesco-Sjögren’s syndrome are related to defects
in a gene crucial for the assembly or secretion of
the chylomicron particles, leading to very low serum
levels of vitamin E.
|
|
|
|

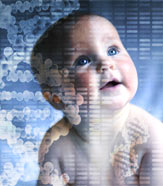

 |
Number 27 VOL. 27 (2), 2024 |
Number 27 VOL. 27 (1), 2024 |
Number 26 Number 26 VOL. 26(2), 2023 All in one |
Number 26 VOL. 26(2), 2023 |
Number 26 VOL. 26, 2023 Supplement |
Number 26 VOL. 26(1), 2023 |
Number 25 VOL. 25(2), 2022 |
Number 25 VOL. 25 (1), 2022 |
Number 24 VOL. 24(2), 2021 |
Number 24 VOL. 24(1), 2021 |
Number 23 VOL. 23(2), 2020 |
Number 22 VOL. 22(2), 2019 |
Number 22 VOL. 22(1), 2019 |
Number 22 VOL. 22, 2019 Supplement |
Number 21 VOL. 21(2), 2018 |
Number 21 VOL. 21 (1), 2018 |
Number 21 VOL. 21, 2018 Supplement |
Number 20 VOL. 20 (2), 2017 |
Number 20 VOL. 20 (1), 2017 |
Number 19 VOL. 19 (2), 2016 |
Number 19 VOL. 19 (1), 2016 |
Number 18 VOL. 18 (2), 2015 |
Number 18 VOL. 18 (1), 2015 |
Number 17 VOL. 17 (2), 2014 |
Number 17 VOL. 17 (1), 2014 |
Number 16 VOL. 16 (2), 2013 |
Number 16 VOL. 16 (1), 2013 |
Number 15 VOL. 15 (2), 2012 |
Number 15 VOL. 15, 2012 Supplement |
Number 15 Vol. 15 (1), 2012 |
Number 14 14 - Vol. 14 (2), 2011 |
Number 14 The 9th Balkan Congress of Medical Genetics |
Number 14 14 - Vol. 14 (1), 2011 |
Number 13 Vol. 13 (2), 2010 |
Number 13 Vol.13 (1), 2010 |
Number 12 Vol.12 (2), 2009 |
Number 12 Vol.12 (1), 2009 |
Number 11 Vol.11 (2),2008 |
Number 11 Vol.11 (1),2008 |
Number 10 Vol.10 (2), 2007 |
Number 10 10 (1),2007 |
Number 9 1&2, 2006 |
Number 9 3&4, 2006 |
Number 8 1&2, 2005 |
Number 8 3&4, 2004 |
Number 7 1&2, 2004 |
Number 6 3&4, 2003 |
Number 6 1&2, 2003 |
Number 5 3&4, 2002 |
Number 5 1&2, 2002 |
Number 4 Vol.3 (4), 2000 |
Number 4 Vol.2 (4), 1999 |
Number 4 Vol.1 (4), 1998 |
Number 4 3&4, 2001 |
Number 4 1&2, 2001 |
Number 3 Vol.3 (3), 2000 |
Number 3 Vol.2 (3), 1999 |
Number 3 Vol.1 (3), 1998 |
Number 2 Vol.3(2), 2000 |
Number 2 Vol.1 (2), 1998 |
Number 2 Vol.2 (2), 1999 |
Number 1 Vol.3 (1), 2000 |
Number 1 Vol.2 (1), 1999 |
Number 1 Vol.1 (1), 1998 |
|
|